Glossary
Actor-Network Theory
Actor-network theory (ANT) was developed in the 1980s. Two of its best-known proponents are the French sociologists Bruno Latour (b. 1947) and Michel Callon (b. 1945) (Seng 2018). According to this theory, instead of focusing solely on humans, non-human actors such as machines, inanimate objects, and microorganisms are also seen as agents. In ANT, humans and non-humans are equal, meaning there is no hierarchy between their actions (Latour 2014, 81). Actors form a network by engaging in social actions with each other. “Social” in this context captures “a particular type of association between hitherto ‘unassociated’ forces” (Latour 2014, 112), which can be both material and semiotic (Seng 2018, 28).
Actor-network theory is an important starting point for interdisciplinary research and offers new perspectives for explaining innovation. In particular, ANT decisively broadens the view of the causes and main influencing factors of innovations. From the perspective of ANT, innovations are created by linking heterogeneous actors into networks and by coordinating their behavior within this framework (Schulz-Schaeffer 2000, 277).
Looking at the work of Anna Dumitriu and Alex May against this background, yeast fungi and bacteria are to be understood less as material than as actors. In Fermenting Futures, yeast is not only an essential “player” in solving environmental problems, and the sedentarization of humans, but also a co-author of several works.
Latour and Pasteur: Yeast as a Player
Yeast plays an interesting role in Bruno Latour’s work, in which he uses it to exemplify the actor-network theory. In Les Microbes. Guerre et paix, suivi de irréductions (The Microbes: War and Peace Followed by Irreductions), Latour raises the question of how Louis Pasteur (1822-1895) arrived at insights such as pasteurization on the one hand and the development of vaccines on the other. Latour parallels Pasteur’s achievements with Tolstoy’s novel War and Peace, using the latter to demonstrate that great discoveries cannot be the result of a single actor (Knorr-Cetina 1985, 578). Consequently, Pasteur’s achievement could be attributed to an “unknown mass” in which the reaction of yeast also played a role (Knorr-Cetina 1985, p. 578).
Aesthetic Cognition
In the Wunderkammer, art and science, collecting and research, were on equal footing. Accordingly, there was no hierarchy between rational and aesthetic cognition; two distinct ways of understanding the world. If rational cognition occurred via the intellect and was based on logical-analytical methods, aesthetic cognition occurred via intuition and sensual perception (Brandstätter 2013, 32-40). With the growing influence of the natural sciences, constantly analyzing and explaining new areas of the world, the balance shifted. Rationalists devalued aesthetic experience, and as a result art also lost importance as a medium for cognition.
The philosopher Alexander Gottlieb Baumgarten (1714-1762) outlined a counterposition to the rationalist view in his writing Aesthetica (1750/58). He argued that the aesthetic approach to the world was “just as capable of producing assured knowledge” as the rational one, “because in aesthetic experience wholeness, coherence, and meaning are (directly) experienced” (Maeder 2017, 62). Art not only provided the visual objects through which experience materialized, it was also symbolically rich in content, expanding beyond its immediate encounter.
In the 20th century, John Dewey (1859-1952) and Nelson Goodman (1906-1998) took up these thoughts anew. According to Dewey, art makes it possible to experience the central moments of everyday life; it succeeds in unifying different and changing experiences. Thus, aesthetic knowledge is more expansive than rational knowledge, and this is precisely what constitutes its contribution to knowledge of the world (Dewey 1980). For Goodman, works of art are ultimately signs that can be interpreted indefinitely. This form of understanding never comes to a conclusion. It is always worth looking again (Bertram 2005, 193).
Allianztechnik
The term “Allianztechnik” (alliance technology) appears in the 37th section of the multi-volume work Das Prinzip Hoffnung (The Principle of Hope) by the German philosopher Ernst Bloch (1885-1977), a publication devoted to “Will, Nature, the Technical Utopias.” The following sections elaborate on what Bloch means by alliance technology, but without using the term explicitly. Bloch described alliance technology as the “co-productivity of a possible subject of nature” (Bloch 1985, 802-807). According to Bloch, the various forces at work in nature cannot be traced back to a single factor. Rather, these forces are based on a chain of mutually influencing factors, amongst which human-made tools and techniques are only one aspect. Here Bloch is indicating that humans are also part of nature. By building houses and shaping their environment, humans create their own second nature that, nonetheless, remains subject to the laws of nature. Therefore, if the work of humans and nature cannot be separated, then every technical development is only a new form in which the forces of nature are expressed. For example, the steam engine is an apparatus that allows the inherent forces of heated water to become visible.
Alliance technology shows that humans cannot impose their will on nature, but that each human development takes place in relation with nature, taking into account the “co-productivity of nature” (Bloch 1985, 802-807). Human creative capacity and natural forces go hand in hand in every development, and with every new technology the question arises as to the consequences for the entire biosphere. How can technologies contribute to a successful life? Bloch’s alliance technology is an attempt to see humans and nature as equal agents. In this context, technological achievements are not necessarily opposed to nature, but their effects must be seen in the overall context of nature.
Anthropocene
In reference to the common ages of the earth until then, the term “Anthropocene” was coined by Paul J. Crutzen in 2002 (1933-2021) to refer to the dawning of a new one, which would be massively influenced by humans.
Together with his colleague Eugene F. Stoermer (1934-2012), he publicized the term and explained the factors that characterize the Anthropocene (Horn 2919,8). Under the influence of man-made technologies, the bio- and noosphere have undergone massive changes. These changes roughly start with industrialization in the 19th century. The effects are increasingly evident in environmental pollution, climate change, overexploitation and loss of resources, and species extinction. In addition, in the course of globalization, species are being displaced and, finally, the atmosphere is becoming increasingly polluted by light, among other things, which in turn has a number of consequences for the entire ecosystem. Since no species exists in isolation, rather there is an interplay of biological as well as non-biological actors, changes to one system inevitably draw those to further systems (Haraway 2015, 159).
After the term Anthropocene is introduced, it finds further differentiation, especially also with regard to the relationship between humans and the environment (Ehlers 2008, 171-228). A distinction is made between a late, a middle, and an early Anthropocene. Donna Haraway et al. illustrates how human-induced changes are linked to capitalist systems and the exploitation of nature that results in the extinction of any retreat. Thus, there is no longer any possibility for individual species to regenerate. (Tsing, 2015). To clarify the complex interconnections responsible for the changes that condition the Anthropocene, Haraway suggests further labels with “Plantationocene,” “Capitalocene,” and “Chthulucene.” (Haraway 2015, 160).
Global warming is only one characteristic of the Anthropocene. As humans increasingly shape their environment and have to deal with the consequences, they are also fundamentally changing their relationship with nature. Nature is no longer nature that has emerged from itself, but a product of man, acting back on him and giving rise to ever new methods of its control.
Art and the Natural Sciences
The demarcation of art as a discipline to be distinguished from the sciences is of comparatively recent date and goes back essentially to the cult of genius of the 18th century and the founding of modern aesthetics by Immanuel Kant (1724-1804) (Kultermann 1987, 117).
The Latin term ars as well as its Greek equivalent technē initially designate a skill that includes craftsmanship as well as artistry. The classification of artes liberales goes back to antiquity, and distinguishes between two groups. The first group includes grammar, rhetoric, and dialectic or logic. The second includes arithmetic, geometry, music and astronomy, and from the early Middle Ages, mechanics and medicine. Opposite the liberal arts were the practical ones, the artes mechanicae, under which, amongst other things, was included the art of building. There was no hard division between art and science, as we understand it today.
With the Renaissance, the relationship between art and science began to be questioned. At the same time, personalities such as Leonardo da Vinci (1452-1519) and Albrecht Dürer (1471-1528) emerged-artists who were also concerned with researching scientific laws, such as perspective, anatomy and proportions.
The concept of art in the modern sense, which considers artistic creation detached from any craftsmanship, appeared primarily with Alexander Gottlieb Baumgarten (1714-1762) and Immanuel Kant. For Baumgarten, art was an expression of sensual cognition. Kant subsequently introduced taste as a category largely detached from understanding, which serves to grasp the beautiful in order to further define art as the purpose-free beautiful (Kant 1993, 77). Decoupled from understanding and purpose, art began to separate from the natural sciences. This divergence persisted until the technological revolution of the mid-20th century. With initiatives such as GRAV – Groupe de recherche d’art visuel at the beginning of the 1960s in Paris, or the experiments carried out in 1966 by artists in the aeronautics center of the Art and Technology Program at the Los Angeles County Museum of Art in California, a rapprochement began to occur (Fiorentini 2010). Since the 1990s, institutions such as the ZKM in Karlsruhe have focused on the complementarity of science and art. Art as science and science as art is now provided a firm framework with artistic research that is also recognized through science funding.
Artistic Research
The term “artistic research” describes an art practice that is primarily concerned with the communication and expansion of knowledge (Vogel 2021, 69). In doing so, artists can address questions with unconventional methodological approaches. Artistic research in contemporary art goes back to the 1960s, for example the cooperation of artists of the Light and Space Movement in the Art and Technology Program with the University of California. Art schools are increasingly offering artistic research as a discipline, and more and more artists are working in laboratories at universities together with teams from the respective disciplines.
Artistic research includes a variety of approaches, including speculative artistic research (Holert 2011, 39). Speculative artistic research can be understood as a thought experiment that takes on an artistic form. It can be a fictional project or one that relates to existing research findings whose utopian or dystopian consequences are acted out in an exemplary way (Catts 2018, 66).
Anna Dumitriu and Alex May’s work moves between the two practices. While Fermenting Futures, for example, can be categorized as artistic research, in Biotechnology from the Blue Flower Dumitriu and May develop a vision of turning back evolutionary change, opening the horizon for broader considerations, in this case, such as how genetic engineering can counteract the loss of biodiversity.
Motivation for Alliances between Art and Science
From the iconic DNA double helix to space photography, we encounter images that serve to illustrate complex content or document scientific findings. Alliances between art and science can be traced historically to the early Middle Ages (Vogel 2021, 54). For a long time, the focus was primarily on the visualization, communication, and illustration of knowledge.
Since the 1990s at the latest, new motivations and methods can be observed in the collaboration between artists and scientists. In this context, artistic creation does not only appear as an instrument to illustrate complex scientific knowledge. Rather, it questions established methods of knowledge production and expands them through new approaches. For example, art links scientific questions with subjective experiences or historical references (Vogel 2021, 54), but also with current social, political, economic, or ethical aspects (Catts 2018, 66). As artistic practice can network with many topics in an open-ended way, productive leaps of meaning can emerge pointing to gaps in previous considerations and generating new thoughts.
BioArt & Transgenic Art
The term “BioArt” was first used in 1981 by Peter Weibel (born 1944) in his essay Biotechnology and Art. Weibel, currently director of the ZKM Karlsruhe, thus captured the connection between art and biology. Today, BioArt refers to art that deals with the molecular biological level of organisms and includes, amongst other things, tissue engineering, i.e. the cultivation of biological tissues.
A special variety of BioArt is Transgenic Art. Transgenics are organisms to which exogenous (i.e. foreign) DNA has been inserted. The recombination of DNA across species boundaries was developed in 1973 by Herbert Wayne Boyer (b. 1936) and Stanley Norman Cohen (b. 1935) and has been a topic in art since the 1980s (Reichle 2005, 92; Reichle 2018). Transgenic Art as a term for this art movement was introduced in 1998 by Brazilian media artist and theorist Eduardo Kac (b. 1962). Transgenic Art is characterized by working with organisms whose genetic code has been altered with the use of molecular biological technologies.
Clearly distinguished from BioArt and Transgenic Art is Artificial Life Art, which works with computer-based simulation models such as algorithms and artificial intelligence (Reichle 2005, 3). It deals with the “bringing to life of the technical” while Transgenic Art deals with the “technification of the living” (Reichle 2005, 189-192).
BioArt and Transgenic Art: Lines of Development
In BioArt and Transgenic Art, two lines of development can be distinguished: one in which the artists themselves apply technologies of molecular biology, and a second in which their goal is to provoke a discourse about these methods without themselves working on modified organisms in the laboratory. New molecular biology tools (for example CRISPR/Cas) always require social processes of negotiation, which are also conducted in BioArt or even initiated by it. In this context, the interests of BioArt artists have shifted over time, as Ingeborg Reichle describes (Reichle 2018, 45). In the early years of this art movement, artists were mainly interested in using the newly developed processes of biotechnology in creative ways. More recently, the climate crisis has been one of the main thematic focuses of BioArt, particularly the extinction of species and the potential of ecological collapse. Anna Dumitriu and Alex May take up these themes in their work Fermenting Futures.
BioArt and Transgenic Art: Reference Positions
There are two major trends in Transgenic Art, whose main representatives are Eduardo Kac (born 1962) and Joe Davis (born 1950). Kac became known worldwide through his work GFP Bunny (http://www.ekac.org/gfpbunny.html). In a laboratory, green fluorescent protein (GFP) was inserted into a rabbit via gene transfer. However, the animal was never handed over to Kac. Nevertheless, the project brought ethical debates about genetic research into the international press. Crucial to this work is its staging, in which Kac makes equal use of references to art and cultural history as well as scientific illustrations. Through works such as GFP Bunny, Kac aims to raise awareness of the fact that interventions in DNA make it possible to “design” new living beings according to aesthetic criteria. He also sees the manipulation of human genes as already within reach: “Being human will mean that the human genome is not a limitation, but our starting point.” (Reichle 2005, 50).
Joe Davis, on the other hand, works directly in the laboratory, and is considered one of the first artists to trade his studio for a lab. Since 1992 Davis has been based at the Massachusetts Institute of Technology in Cambridge, where he first became artist-in-residence and later associate researcher in the laboratory of biology professor Alexander Rich. Davis’ research is on “coding and sequencing problems of the genetic code” (Reichle 2005, 80). His work Microvenus (1986) shows how the Germanic symbol for life and the earth is translated first into a binary code and then into a DNA sequence (https://www.clotmag.com/biomedia/joe-davis; Reichle 20055, 83). Another example of Transgenic art is the Tissue Culture and Art Project, a collaboration between Australian artists Oron Catts and Ionat Zurr. Catts and Zurr often work with living cell material, taking the laboratory situation directly into the exhibition (figures: https://tcaproject.net/)
Anna Dumitriu and Alex May, like Joe Davis, work in the lab themselves, delving as deeply into the field of biotechnology as Davis does. Ingeborg Reichle wrote in her 2003 dissertation that Davis was perhaps the only true “artist researcher” (Reichle 2005, 112). However, from today’s perspective, this statement is no longer valid, since Dumitriu and May also develop the complex molecular biological content of their works themselves while in close exchange with the scientific community. At the same time, they make use of a rich system of references to art and cultural history, which is reminiscent of Kac’s way of working. Dumitriu and May thus combine characteristics of the two major currents of Transgenic Art.
Through Dumitriu and May’s working methods and the close cooperation with scientists, a rapprochement between art and science occurs. At the same time, the works enable a discourse between research and the public, taking on a mediating role, and inviting viewers to discover their complex scientific and historical backgrounds. Like Kac, Dumitriu and May thus succeed in sensitizing viewers to the ethical issues involved in molecular biology without losing sight of its opportunities.
Biotechnology from the Blue Flower
Biotechnology from the Blue Flower was developed in collaboration with the European research project CHIC. It focuses on chicory (Chicorium intybus). In the context of their artistic work, Dumitriu and May have dealt with the gene editing methods used in plant breeding techniques, the morphology of the plant itself, and its historical-mythological dimensions. The result is an installation that includes a 3D printed sculpture, a video generated from an edited version of the chicory genome, and an app.
CHIC-PROJEKT
The CHIC project focuses on the development of new chicory varieties. The starting points are two natural substances produced by the plant: Inulin and terpenes.
Inulin is a prebiotic, digestive fiber and is also used as a sugar substitute. One of the project goals is to breed a chicory that produces more and better inulin. To do this, the genes responsible for producing inulin are modified using the CRISPR/Cas method.
Terpenes cause the bitter taste of chicory, but they also have medicinally relevant properties. For example, they can have an anti-inflammatory or analgesic effect. The CHIC project is therefore investigating, on the one hand, which medicinally useful terpenes chicory possesses and which genes are responsible for the synthesis of the terpenes. On the other hand, research is being carried out to determine which of these genes need to be switched off in order to prevent the production of bitter substances and make the plant more useful as a sweetener. The CHIC project thus combines gene editing with research into the chicory genome.
Material Research
Dumitriu and May started their artistic exploration of chicory by researching its structure and morphology. For example, they stained sections through the root and were thus able to visualize the terpenes stored in it. They scanned a chicory plant, including the root, using a technique called photogrammetry to create a virtual 3D model of chicory that enabled a visual journey through its interior.
Through this approach, Dumitriu and May literally take a new look at the plant, uncovering its aesthetic potential. The strategy of seeing beauty in banal objects that are often overlooked or inconspicuous to the aesthetic eye is also found in Bioarcheology of Yeast, a part of the Fermenting Futures group of works. In the lab as artists in residence, Dumitriu and May also genetically edited chicory protoplasts with CRISPR/Cas, prompting further contextualization.
The Blue Flower
The chicory made a career for itself as a model for the blue flower, the central symbol of Romanticism. In his novel fragment Heinrich von Ofterdingen, Novalis (1772-1801) set a literary monument to it and made it the symbol of the Romantic longing for new horizons of consciousness and experience (Malaguti 2005, 207-225).
Romanticism was a reaction to the Industrial Revolution, man’s alienation from nature and its disenchantment by (natural) scientific explicability. By addressing the historical symbolic character of chicory, Dumitriu and May take up the question of our relationship to nature, relevant then as now. What meaning will “nature” have in the future? How will man-made changes be distinguished from natural processes? Is even switching off a gene already a manipulation of nature? The answer to these questions will decisively shape the acceptance of genetic engineering methods and the evaluation of their opportunities and risks.
Goethe’s “Urpflanze”
In addition to the legendary significance of chicory, another historical reference is Johann Wolfgang von Goethe’s (1749-1832) idea of the “Urpflanze”(primordial plant). In his theory of metamorphosis, he equates the primordial plant with an archetype that underlies all plants and from which they emerged with their various expressions. Goethe gained his idea of the primordial plant through intensive studies of nature, which finally resulted in his work Versuch die Metamorphose der Pflanzen zu erklären (Attempt to explain the metamorphosis of plants) in 1790. In it, Goethe explains how different forms of a single plant – for example the stem, sepals and petals – can be understood as metamorphoses of the same basic organ (Goethe 1980, 78). A few years earlier he notes, “Hypothesis Everything is leaf, and through this simplicity the greatest manifoldness becomes possible.” (Kuhn 1977, 58). Everything is leaf – this can also be said of the process of genetic engineering plant breeding, since in the laboratory the entire plant with all its different organs is drawn from a single cell – the protoplasts.
Also, the video alludes to the notion of the primordial plant. It shows the genome of the chicory, from which all – according to a current hypothesis acquired in the course of evolution – repeating DNA sequences have been removed. Can this artistically speculative gene knockout be used to turn back evolution and restore the chicory’s original form?
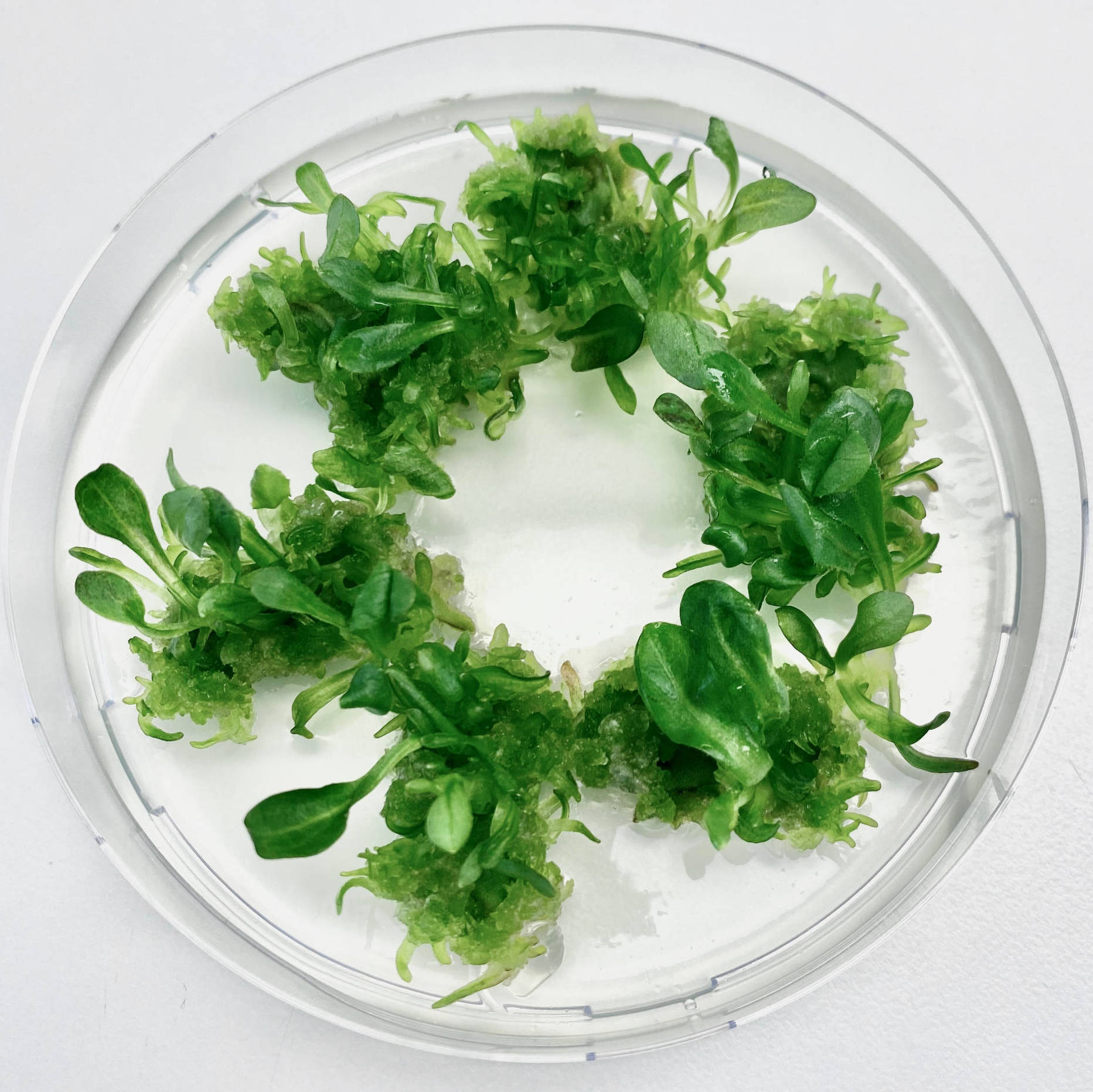
Photo: Anna Dumitriu and Alex May
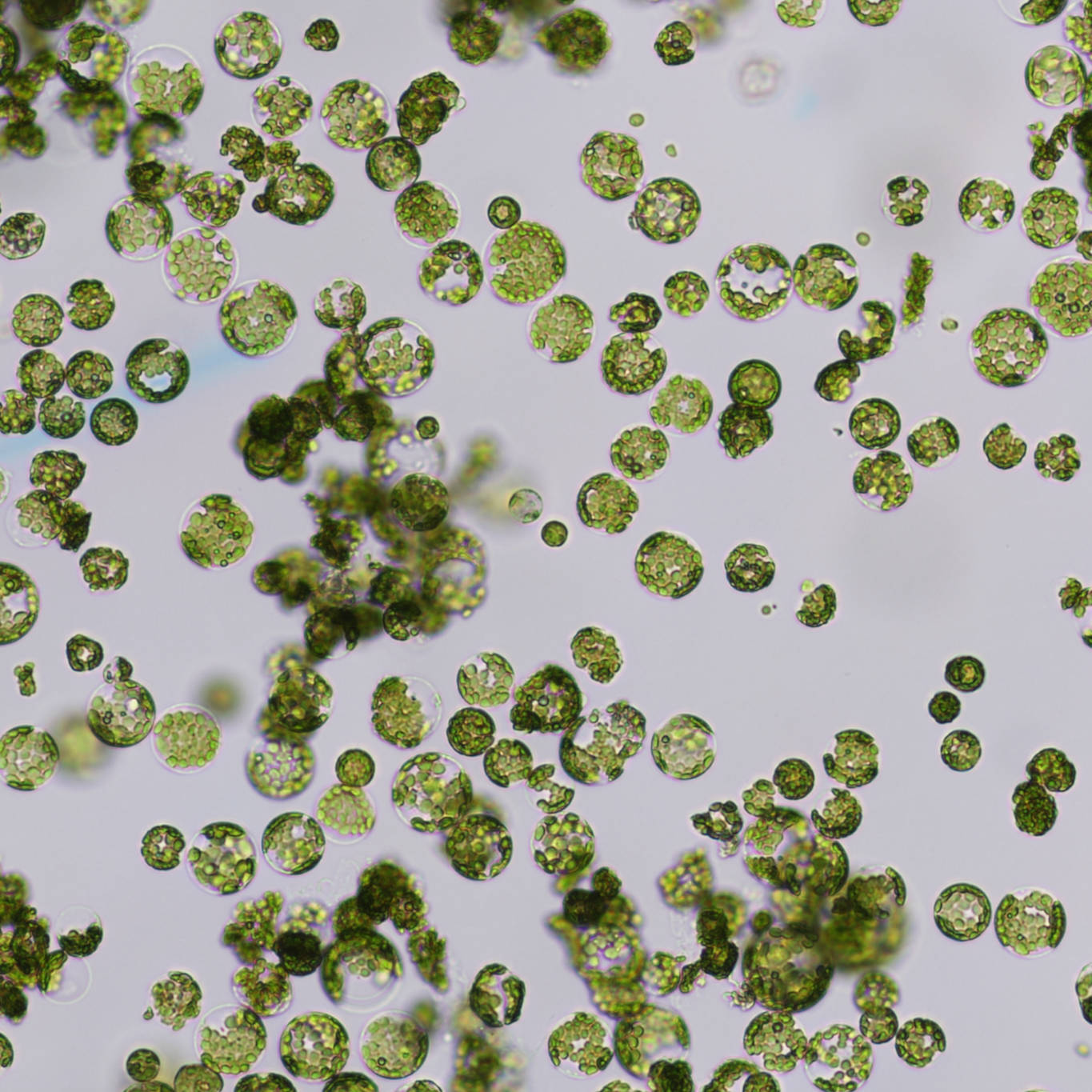
Photo: Anna Dumitriu and Alex May
Biotechnology from the Blue Flower – Sculpture and App
The sculpture is a 3D print of a chicory based on high-resolution photogrammetry scans of the plant clones that CHIC project researchers are working with. It also contains relics of CRISPR-modified plant material from the lab. Thus, the categories natural – artificial and real – digital already interpenetrate, and this is also visible in the form of the sculpture itself. Some of its components represent a realistic image of chicory, while some seem to show glitches – errors in production- and thus reveal themselves to be simulations of nature. The simulation of nature is taken up with the app, as it uses augmented reality to allow chicory to be planted at any location. The narrow legal limits that exist for the agricultural use of genetically bred plants – and also for the possibility of exhibiting genetically modified organisms in an art context – are thus transgressed, at least in virtual space.
Cabinet of Curiosities
Artfully formed objects, whether created by nature or by man, aroused a passion for collecting early on and found their way into specially created cabinets. Such compilations, known as chambers of art and curiosities, brought together objects from art and nature in order to place them in a context, arranged according to themes. The four elements were used as a criterion of order, often combined with the cardinal points and the known regions of the world. The first known chambers of art and curiosities were found at princely courts, such as that of Archduke Ferdinand II. (1529-1595) at Ambras Castle in Tyrol or Rudolf II. (1552-1612) in Prague Castle. The chambers were intended to reflect the richness of the universe in a microcosm and to illustrate connections between nature, art, science, and technology (Bredekamp 2012, 70). Accordingly, they were subdivided into Naturalia, Artificalia, Antiquitates, Exotica, Mirabilia, and Scientifica without hierarchy. The classifications of objects were based less on a scientific than on a purely associative basis.
At the same time, the chambers of art and curiosities also reflected the perception of the world typical of the time, which placed the earth at the center. Often, this was underlined by a corresponding perspective spatial design.
The forerunners of the chambers of art and curiosities can be seen as the Museien, the temples of antiquity intended as seats of the Muses. The designation studiolo, common in 14th-century Italy, underscores their function as study cabinets that served scholarly exchange, but also the demonstration of claims to power and dominion.
Holistic vs. Reductionist View of the World
The chambers of art and curiosities (Wunderkammern) were determined by a holistic view of the world, in which everything was interrelated. This was countered by a reductionist view which came to the fore at the latest in Renaissance. The reductionist view attributed a cause to every effect, which could be traced back to the smallest unit via a chain of causalities. The order of the chambers of art and curiosities gave way to special collections. Natural and art objects found their way into natural history and art museums separately from each other. The striving for universal knowledge was replaced by specialization in one field, guided by the idea that each field could be understood in isolation from others.
With regard to the cooperation of art and science, the chamber of art and curiosities appears as a historical precursor. Not only did the disciplines stand on equal footing here, but the spatial proximity also made it possible to discover previously unseen relationships between objects (Brakensiek 2006, 7). Another parallel emerges when considering the boundaries between objects of art and nature: they were fluid in the chambers of art and curiosities – an aspect around which BioArt also revolves. After all, the “Wunderkammer” was not only a collection space, but also a laboratory and a place for exchanging knowledge (Bredekamp 2012, 53). Against this background, contemporary laboratories in which artists work may be seen as (modern) chambers of art and curiosities, too.
Chicory
The chicory plant (Cichorium intybus) is often found on dry and nutrient-rich roadsides. It is mainly found in Central Europe and reaches a height of up to 140 cm. It blooms from June to October; its blue, star-shaped flower heads depend on the position of the sun, opening in the morning and closing in the evening. Chicory is a member of the composite family (Asteraceae). It is closely related to vegetables such as witloof, radicchio or endive. Already in ancient times it was used as a medicinal plant, for example, for digestive disorders or to stimulate the liver, gall bladder and spleen.
The special feature of chicory is its thick, spindle-shaped root. This is not only used as a coffee substitute. In it, the plant also accumulates inulin. This substance is already being commercially exploited today as a dietary fiber and sweetener in foods, and as a prebiotic due to the digestive effect of inulin.
Cooperative Research Networks
The Kunst- und Wunderkammer, established at courts of the European aristocracy since the 16th century, have much in common with the ways that virtual networks can be continually restructured and reknit. If the chambers of art and curiosities contain physically tangible objects, the Internet contains virtual objects whose substance is a binary storage code (Brakensiek 2006, 5). Yet in both spaces—the real and the virtual—juxtaposition forces the formation of associations.
All objects have several meanings, which become apparent through various combinations with other objects. In the historical Kunst- und Wunderkammer, the position of the objects was decided according to a certain systematic approach, remaining spatially static. However, the order and groups in which individual objects were ultimately placed, was flexible. In this way subjective order triumphed over alphanumeric systems that later became common in museums, libraries, and institutionalized collection contexts. On the other hand, digital collections, especially when they combine to form research networks, leave assignments largely open. Associations between objects are made anew with each unique site visit, and with regard to the respective individual search terms used (Brakensiek 2006, 12).
CRISPR/Cas
CRISPR/Cas and GMO are abbreviations for a genetic method and genetically modified organisms. GEO is also occasionally used, meaning Genetically Engineered Organism. Genetically modified emphasizes that it is a targeted access to the gene code, clearly expressing that the process is different from conventional methods of cross-breeding, random mutation and recombination.
The CRISPR/Cas system is a method used to modify organisms. Specifically, it allows the targeted removal, insertion or replacement of specific DNA sequences in the genome. Genes can be inserted both from the species itself and from other species. These include genetically modified bacteria, which have been used for insulin preparations since 1999, as well as lactic acid bacteria, which affect the fermentation of dairy products. Transgenic yeasts, in turn, can be used to produce low-calorie beers (Wankerl, 2013, 48).
By interrupting the base sequence, genes can also be specifically switched off. Depending on the organism, a distinction is made between green, red and white genetic engineering.
CRISPR/Cas was discovered in bacteria as an organism’s own protective mechanism against viruses. The system is composed of two parts. CRISPR – one part – stands as an abbreviation for Cluster Regularly Interspaced Short Palindromic Repeats and refers to sections of repetitive DNA. Foreign DNA, for example from viruses, is stored in these sections. CRISPR thus serves as a storage site or cellular memory. Together with the other part, the enzyme Cas (CRISPR associated), it is a central part of the bacterial immune system.
Using CRISPR sequences, the Cas enzyme recognizes viral DNA that has entered the bacterium. This results in Cas cutting the recognized DNA, destroying the invader’s genome and thus avoiding viral infection. Because of this reaction, the CRISPR/Cas system is also commonly referred to as gene scissors. In biotechnology, this system enjoys great popularity. By modifying the CRISPR sequences, one can have the Cas enzyme cut genomes at any desired location in any living organism (Jinek et al. 2012).
There are several types of Cas enzymes, which are classified into different groups. For example, the commonly used Cas9 enzyme belongs to a group of Cas enzymes that are capable of cutting DNA. Other Cas enzymes, on the other hand, only cut RNA.
CRISPR in Laboratory Practice
CRISPR/Cas refers to both the enzyme and the procedure that can be used to intervene on the base sequence of a DNA. Colloquially, CRISPR/Cas is known as gene scissors.
CRISPR stands for “Cluster Regularly Interspaced Short Palindromic Repeats” and refers to sections of repeated DNA, which in turn provide the prerequisite for various modifications. When CRISPR/Cas is used, parts of the genome can be removed, inserted or exchanged. In this way, for example, genes with undesirable traits can be specifically eliminated or genes from extraneous organisms can be introduced.
CRISPR/Cas is also being used at Goethe University Frankfurt am Main. During a visit to the Institute for Molecular Bio Sciences, where, among other things, research is conducted at yeast, Professor Dr. Eckhard Boles and his team explain how the gene scissors work and provide insights into laboratory practice (see video: Die Genschere im Laboreinsatz).
Development of a Modern Concept of Nature
In the wake of the theory of evolution, nature was understood as an open system in every respect. The development of cybernetics plays a decisive role in the modern understanding of nature. Cybernetics as a scientific discipline of the control and regulation of dynamic systems goes back primarily to Norbert Wiener (1894-1964). Wiener brought together mathematicians, engineers and neuroscientists to investigate the parallels between the brain and the computer and to make the findings usable for control techniques.
Analogous to the mechanistic worldview, functional processes of biological organisms were now transferred to machines. According to cybernetics, both humans and machines function via complex and dynamic control circuits and are thus information-processing systems, independent of their respective material composition.
Cybernetics eventually opened the door to Artificial Life Art. Its protagonists were concerned with “making machines ‘come alive’ in their practices and discourses, synthesizing life and generating artificial life in the computer” (Reichle 2005, 116). As in BioArt, which developed in parallel, the artist becomes a creator who no longer copies but creates a new.
DNA as a storage medium
DNA is not only capable of storing human genetic information. For researchers, DNA as a storage medium for any kind of data is an interesting topic because it is more robust and durable than many storage media. While a CD can only hold data for about 30 years, DNA is said to be able to store the data it contains for hundreds of years. The American artist Joe Davis, one of the pioneers of BioArt, worked with DNA as a storage medium as early as 1986. With Microvenus, he created an image that was encoded into a genetically modified bacterium. The image shows an ancient Germanic symbol, a rune, which symbolizes life and the female earth (Davis 1996, 70-74). Davis was interested in exploring communication possibilities with extraterrestrial intelligences, which NASA has been doing for decades (Davis 1996, 70-74).
Ecocritical Art
Briefly defined, ecocriticism investigates problems of ecological connectivity, sustainability, and justice through the lens of culture (Braddock 2009). Accordingly, ecocritical art refers to art forms that address ecological problems and their social implications. In doing so, ideas of nature, society, and ecology – especially the role of humans as actors – are fundamentally questioned (Martin 2015, 5).
While some artistic positions only focus on picking up, analyzing, and documenting problems and their effects, other artists also deal with considerations of what solutions can be pointed out for the individual problems. These approaches to solutions can be based, for example – as in the case of Dumitriu or May – on scientifically founded approaches and potentially realizable possibilities, or they can also be speculative-utopian. The artistic strategies thus often imply an intensive, cross-disciplinary collaboration.
The artistic forms of expression are broad, ranging from more classical media such as photography to participatory or activist-performative processes (Rapp, de Lutz 2021, 56).
Encoding language in DNA
In order to translate language, a complex sequence of 26 letters and special characters, into DNA, an equally complex sequence of 4 nucleotides abbreviated A, T, G, and C, a number of steps are necessary. For the translation, Anna Dumitriu used the ASCII code (American Standard Code for Information Interchange), which is based on the binary system (also called dual system). In the ASCII code, each letter is assigned a specific 7-digit combination of the binary elements 0 and 1.
M becomes 1001101.
A becomes 1100001.
k becomes 1101011.
And e becomes 1100101.
Besides the binary system, which only knows the digits 0 and 1, there are other systems. The most common number system for us is the decimal system, consisting of the digits 0, 1, 2, 3, 4, 5, 6, 7, 8 and 9. The genetic information stored in DNA is based on the arrangement of four different bases: Adenine (A), Thymine (T), Guanine (G) and Cytosine (C). If we assign a digit to each of these bases (A=0, T=1, G=2 and C=3), we see that genetic information is stored in the form of a quaternary system, a system of 4 digits (0, 1, 2, 3). If we now translate the letters within ASCII into a quaternary system, we obtain new numbers in each case, which now consist of four digits or four DNA bases.
That means:
(M) 1001101 becomes 1031 resp. TACT
(a) 1100001 becomes 1201 or TGAT
(k) 1101011 becomes 1223 or TGGC
(e) 1100101 becomes 1211 or TGTT
The DNA base sequence TACT TGAT TGGC TGTT thus encodes the word “make”.
This DNA sequence is created chemically with the help of the so-called phosphoramidite synthesis. Modified nucleotides are linked one after the other in the intended sequence via chemical reactions until the desired sequence is synthesized. After a specific region in the DNA has been excised, e.g. with the “gene scissors”, the synthesized DNA can be placed exactly at this empty site via homologous recombination, a natural DNA repair mechanism.
Ethics and BioArt
More than other art forms, BioArt invokes ethical questions in addition to aesthetic ones, but this is due less to the actual artworks and more to their reference to practices in biological research. Ethics is highly relevant to the treatment of living organisms, and it is at the heart of biological research. In philosophy, ethics reflects on ways of acting that are appropriate and right. Aesthetics, on the other hand, is concerned with processes of perception and sensory cognition and is most relevant to artistic expression; ethical considerations do not play a role. Although ethics and aesthetics do not have an immediate interface, they meet in the works of BioArt (Vaage 2016, 88). The reason for this overlap is BioArt’s preoccupation with living material: microorganisms such as bacteria, viruses, and fungi, but also plants and animals. In living material, the possibilities and implications of biotechnology are evident and at the same time placed for our disposal.
In the context of art, it is not only the modification of living material that is discussed from an ethical point of view, but also the fact that certain materials become the subject of artistic work. For example, Steve Kurtz (b. 1958) of the Critical Art Ensemble triggered discussions about the safety of handling biological material when it became known that the artist was in possession of microorganisms that could be used as biological weapons (Yetisen 2015, 730).
The ethical assessment of BioArt is now often held to the same standards as biotechnological practices themselves. As a result, the question of what a work of art should actually accomplish in the face of provocative and socially controversial content has taken a backseat. If the artwork is repulsive enough, the implicit criticism is easily understood. However, if it is aesthetically pleasing, the artwork may also become the subject of intensified critique, as it is then assumed that it does not comprehensively consider the full dimension of biotechnological possibilities, perhaps even glossing over them.
Ethics and Genetics
Works of art that address genetic modifications are often subject to particularly harsh criticism. Even when interventions in DNA are justified through advantages to ecology, health, or other areas of application, the long-term consequences of these interventions remain difficult to assess. For example, how the effects of genetic modification will develop in the course of a hereditary succession is impossible to determine.
Microbiological research, which strives for knowledge and wants to account for the possibilities of genetic changes, is not deontologically oriented; i.e. it does not consider its possible consequences. Utilitarian considerations (i.e. weighing up the cost-benefit ratio) are used as an assessment standard for judging whether a particular research project should be carried out and approved or not.
The questions posed to biotechnology are countered by the aesthetic concerns of art. Works with amoral content will not necessarily create moral awareness and may consequently fail to garner attention for certain issues (Vaage 2016, 91). However, by presenting the facts about genetic engineering, artists draw attention to consequences that may not have been discussed before. Artists can highlight moral and ethical concerns and influence decisions (Vaage 2016, 102).
Fermenting Futures
Fermenting Futures is inspired by a number of recent scientific findings on yeast research and was developed in close collaboration with the Institute of Microbiology and Microbial Biotechnology at the University of Natural Resources and Life Sciences (BOKU), Vienna, and the Austrian Center of Industrial Biotechnology (acib). The project by Anna Dumitriu and Alex May consists of four works, each exploring different stories around yeast and investigating the potential of these often unnoticed micro-organisms. From an artistic perspective, collaborating on yeast research is also about transforming and recontextualizing ideas and materials (Schachinger 2020).
Yeast research at BOKU
Yeast is one of the most studied organisms in molecular biology. The scope of their bioeconomic applications is wide, ranging from the manufacture of biofuels to drug production.
In 2015, the team led by Prof. Dr. Diethard Mattanovich succeeded in explaining the metabolic pathway of the yeast species Pichia pastoris. Pichia pastoris is a non-fermenting yeasts (which means it cannot be used for baking bread), but can metabolize methanol (BOKU 2015). Uncovering this mechanism forms the basis for enabling yeast to metabolize carbon dioxide (Dumitriu et al 2021). This was achieved in 2019 by a genetic modification in which eight genes from plants, bacteria, and other yeast species were inserted into Pichia pastoris. At the same time, three genes were switched off. Therefore the modified yeast now uses carbon dioxide for its metabolism instead of methanol and has the potential to reduce CO2 emissions. In another research project, the originally non-fermenting Pichia pastoris was made to ferment by gene editing.
At the same time, a different yeast species, Saccharomyces cerevisiae, was genetically modified to produce lactic acid. Lactic acid is the raw material for polylactide (PLA) – a biodegradable plastic.
Yeast
In biology, the term yeast does not describe a monophyletic group (group that arose from one ancestor) like, for example, mammals, but rather a plethora of different organisms that simply share a certain characteristic: All yeasts are unicellular fungi that reproduce asexually by budding from a cell.
Yeast is found almost everywhere and can be utilized in numerous different applications. Live yeast is classically used in bread baking or beer brewing, but it can also serve as a probiotic. Non-live yeast is often used as a flavor enhancer or as a highly nutritional food additive.
Fermenting Futures
Fermenting Futures brings together two research projects. BOKU researchers succeeded in combining the carbon capturing properties of a modified Pichia pastoris and the lactic acid producing capabilities of a modified Saccharomyces cerevisiae: The result is a Pichia pastoris “super yeast” that can absorb carbon dioxide and release lactic acid as a basic material for manufacture of biodegradable plastic PLA. This simultaneously tackles two environmental challenges at once – CO2 reduction and plastic pollution (Dumitriu et al 2021).
In their artwork, Dumitriu and May illustrate what Gotthold Ephraim Lessing called the “fertile moment” of this complex process. On view is a bubbling liquid-filled, large glass vessel with silicone tubes protruding from its neck. The installation alludes to the fermentation process of the yeast, which, as a genetically modified organism, is not allowed to leave the laboratory alive for safety reasons. Therefore the yeast floating in the vessel has been killed prior to exhibition. The vessel is positioned on a pedestal made of horse chestnut wood, indicating the natural habitat of Pichia pastoris. It is commonly found on horse chestnut trees and feeds on their sap.
3D prints of based on photogrammetry scans of yeast cultures swarm across the vessel. These are made of PLA. One small white yeast form is made of PLA of the lactic acid produced by the gene-modified yeasts P. pastoris and S. cerevisae. The production quantities are still extremely small, but the research team is working on increasing the tolerance of the yeast to the cell-toxic lactic acid through directed evolution in order to increase production.
Culture
Culture shows architectural models surrounded by a layer of earth. The interiors of the models seem lived-in and some even have electricity and television.
The exterior of the model is covered with bread crumbs. This bread was baked with a fermenting Pichia pastoris yeast developed at BOKU, which can serve as baker’s yeast thanks to genetic modification.
The models and their interiors represent the development of culture since humans first started to put roots down in the Neolithic period. It is suggested that yeast played an important role in this process, enabling the baking of bread and the brewing of beer. Earth also refers to agriculture and the cultivation of grain. It is, both figuratively and literally, the top soil on which civilization is founded: a thin, fragile layer on and with which a constantly growing world population must be nourished.
With Culture, Dumitriu and May achieve a significant change of perspective. Not humans, but a small microorganism becomes a ‘player’ that will perhaps determine our fate in the future just as it did thousands of years ago.
Lactate Dehydrogenase Gene Duet
A sound composition complements the exhibition. Its starting point is the DNA base sequence of the lactate dehydrogenase gene, which is responsible for the production of lactic acid.
Bio-archeology of Yeast
Yeast as a non-human agent is also the focus of the sculpture series Bio-archeology of Yeast. The work is based on 3D photogrammetry scans of colonies of black yeasts. This yeast belongs to an extremophile species, which has adapted to extreme environmental conditions that are generally considered hostile to life. The sculptures are cast in building materials that black yeast likes to colonize. The casts are hand-painted based on the original hues of the black yeast, which actually occur in varying shades such as pink, orange or black. Finally, some of the original small colonies of yeast that were scanned the laboratory were applied to the sculptures.
By revealing the sculptural potential of the yeast fungus, which in other contexts is perceived as a nuisance or even a pest, Dumitriu and May highlight its aesthetic qualities. Decay as a negatively connoted and usually even dangerous process in works of art is re-evaluated as a process of artistically productive material transformation.
Pigments
Pigments investigates another natural property of yeast, namely its ability to produce pigments. For example, yeasts protect themselves from sunlight by producing melanin – a mechanism we also know from human skin. With their textile works, which are dyed with pigments from microorganisms, Dumitriu and May want to stimulate discussion about alternatives to chemical dyes. These could be used both in the fashion industry and in food production.
Genetic Engineering versus Conventional Mutation Breeding
Plants modified using modern genetic engineering methods such as the CRISPR/Cas are not permitted as agricultural crops in many countries around the world. However, there are also conventional ways of introducing useful mutations into the genome and thus generating plants with modified properties. For this purpose, external influences are used which can artificially increase the number of naturally occurring mutations. Such influencing factors, so-called mutagens, can be chemical substances (such as nitrosamines or asbestos), high-energy radiation (such as X-rays and UV radiation) or biological organisms (for example viruses). In modern plant breeding, seeds are exposed to these mutagens for a period of time, and the resulting plants are then tested for their properties (for example, fruit shape and taste, growth behavior, response to various stress situations). In contrast to plants modified with CRISPR/Cas, these plants are approved for agricultural use in most countries, as no artificial introduction of foreign DNA into the plant organism takes place here. However, the major disadvantage of this method is that the mutations induced are uncontrolled. As a result, undesirable mutations occur in addition to beneficial ones.
Genetic Research – Historical Outline
Classical Genetics: Gregor Johann Mendel
Genetic sequences, i.e. the transmission of traits through predispositions fixed in the organism, have been known for a long time. In continuous research, the exact relationships between hereditary code and traits were then gradually explored and revealed.
After Charles Darwin (1809-1882) had described in a first version in 1838 the emergence and change of biological species as an evolutionary process in which the dominant trait prevails in each case, the Augustinian monk Gregor Johann Mendel (1822-1884) turned specifically to the transmission of phenotypic traits by cross-breeding. Between 1856 and 1865, Mendel tested various crosses on pea plants and evaluated them statistically. Since the pea plant expressed different traits, such as purple and white flowers or green and yellow seeds, the transmission of traits could be traced by Mendel performing pollination by hand, i.e., transferring the pollen of one variety to the stigma of the other. The method of crossing developed by Mendel was subsequently applied to crops in order to propagate their seeds and successively improve the variety.
Molecular Genetics
Classical genetics, which includes the rules established by Mendel, was replaced by molecular genetics around the middle of the 20th century. The focus is now on the molecular basis of heredity – DNA and RNA.
The findings that and how molecular structure and hereditary information are interrelated, developed successively, beginning with the isolation of nuclein from the cell nucleus, the discovery of nucleic acid, and culminating in DNA sequencing in 1975, which led to the sequencing of the first eukaryotic genome of Saccharomyces cerevisiae, baker’s yeast, in 1993.
Eukaryotes refer to cells with a nucleus and rich compartmentalization, in contrast to prokaryotes, cellular organisms that, like bacteria, lack a nucleus.
Stages in the Development of Molecular Genetics
As early as 1943, Barbara McClintock (1902-1992) identified in maize the transposon, a DNA segment in the genome that changes its position, thus refuting the idea that the genome is constant. She was awarded the Nobel Prize for her discovery in 1983.
In the 1980s, Richard A. Jorgenson (*1951) discovered RNA interference. RNA interference (RNAi) refers to the suppression of a specific sequence of a genetic disposition induced by RNA molecules. It occurs due to the double strand of RNA responsible for translation and transcription. For developing a method to specifically access RNA interference and thus specifically silence genes, Andrew Zachary Fire and Craig C. Mello were awarded the Nobel Prize in Medicine in 2006.
In 1987, Yoshizumi Ishino and his colleagues found repetitive DNA segments in Escherichia coli K12, commonly referred to as CRSPR. The term CRISPR was first used by Ruud Jansen and his colleagues in 2002.
In 2012, Jennifer Doudna and Emmanuelle Charpentier published their article A programmable dual-RNA-guided DNA endonuclease in adaptive bacterial immunity in the journal Science, describing how specific DNA areas can be precisely targeted and cut.
Feng Zhang of the Broad Institute of the Massachusetts Institute of Technology (MIT) first used CRISPR/Cas9 in mice and human cells in 2013. In 2018, He Jiankui claimed to have used the technique in human embryos after artificial insemination, sparking worldwide debate. Because the changes occur in the embryo, it affects egg and sperm cells and consequently is passed down through generations. The unforeseeable consequences were generally criticized as unjustifiable.
Hypertext
Through the Internet, a new form of knowledge structuring and appropriation is coming to life (Brakensiek 2006,12). Virtual space accommodates many forms of knowledge and information that can be linked arbitrarily through hypertext functions. Since hypertext is ordered non-linearly, linking can occur in any direction and tends to occur throughout digital space. The hypertext structure, in this case comparable to the ordering of the Wunderkammer, already determines linkages. However, since the possibilities for linking and expansion in the Internet are essentially unlimited, the information collected in the network tends toward a wide dispersion of the source material.
Vannevar Bush hinted at similar possibilities in 1945, when he envisioned a machine called Memex “which was intended to expand human memory while helping to develop and expand its associative capacity” (Brakensiek 2006, 7). In the 1960s, Theodor Holm Belson followed with Xanadu, which he described as a universal system for managing knowledge information delivery. In anticipation of the Internet, Xanadu would make all the materials necessary to develop any form of knowledge available electronically. These materials would be in the form of images and texts, and be independent of location (Brakensiek 2006, 7).
Imitation of Nature
Nature is derived from the Latin natura, which corresponds to the early Greek physis. The term denotes the entire process of becoming, growing, blossoming and rising, as well as the innate characteristics of a being.
Until the 17th century, artistic creation was characterized by the imitation of nature. Nature was both a model and a source of inspiration, and art sought to mimetically capture the natural world. The aim was to perfectly duplicate nature, equating its productive power with the power of artistic creation. (Rosen 2003).
Inulin
Inulin is a mixture of various polysaccharides, which are made up of different combinations of up to 100 fruit sugars (fructoses) arranged in chains. Due to its large molecular size, inulin, as found for example in chicory root, is frequently used in food production as a dietary fiber with a prebiotic effect on the intestinal flora. In particular, the group of bifidobacteria can metabolize these complex polysaccharides to short-chain fatty acids, in contrast to humans, because they possess the necessary degradation enzymes (inulinases). Since inulin cannot be absorbed through the small intestine and therefore does not affect blood sugar levels, it is also often used as a starch substitute in the treatment of diabetes patients.
Make Do and Mend
This group of works was created in 2017 to commemorate the 75th anniversary of the first use of penicillin on a patient in 1941. The centerpiece is a patched women’s suit from that period. The patches, like the textile works, contain bacterial material modified with gene editing. Following on from the rationing of scarce goods such as clothing during World War II, Anna Dumitriu creates a link to the issue of antibiotic resistance, as the over-use of these lifesaving medicines has led to bacteria evolving the ability to resist them.
FEAT Residency
Make Do and Mend was created as part of a FEAT (Future Emerging Art and Technology) residency program. Here, Anna Dumitriu collaborated with members of the MRG-GRammar consortium at research institutions in Israel and the United Kingdom. The MRG-GRammar project investigates gene regulation and the interaction between so-called “enhancer genes” and those responsible for the production of proteins. The research results are of great importance for the development of personalized DNA therapies, among other things.
Slogan Make Do and Mend
The slogan “Make Do and Mend,” promoted during World War II, was intended to encourage British women to repair clothing during the rationing period of the war, thereby saving resources (Dumitriu). The motif of mending, which is addressed here, appears twice in the work and refers on the one hand to the visible patching of the clothing, and on the other hand to the invisible artistic gene-editing intervention. Thus, the holes of the women’s suit are plugged and mended with silk. The silk is treated with E. coli bacteria grown on a dye-containing agar and thus could dye the fabric pink (Breedlove 2019, 198-199).
From the genome of the E. coli bacteria and by using CRISPR/Cas, Dumitriu removed an ampicillin antibiotic resistance gene that had previously been inserted into the bacterium for research purposes. Ampicillin is part of the penicillin group. By removing the resistance gene, Dumitriu simulated a condition the bacterium must have had before 1941, i.e. before antibiotics had been developed. The artist then repaired the “defect” using a technique called “homologous recombination” by inserting a synthetically produced DNA fragment. The fragment contains – translated into the four DNA bases – the slogan “Make Do and Mend” (Medina 2019, 317-318).
The textile works belonging to the group of works are also dyed with gene-edited bacteria, again emphasizing the central process of repair.
Manual Work
Sewing, embroidery and mending – in Make Do and Mend, the first thing that jumps out is the use of typically female connotated needlework techniques. The small toy sewing machine (owned and played with by the artist’s mother during WWII) takes up the idea of a rather simple, almost old-fashioned production process and thus reinforces the impression of a low-tech aesthetic. However, this contrasts strongly with the highly complex and modern processes of genome editing that were used in the development of the work in the laboratory. High-tech and low-tech, manual labor and science, perhaps even the clichés of feminine (manual labor) and masculine (research in the laboratory) work are hereby juxtaposed. Yet despite their apparent opposites, they touch each other: the one illustrates the other, since in both fields we speak of activities such as cutting, patching, or plugging holes. According to Anna Dumitriu, both types of work are tricky and require patience, care and diligence – or, as Annick Bureaud aptly puts it: Science is manual labor.
Controlled Commodity
Sewn onto the suit is the British Board of Trade logo CC41, an abbreviation for Controlled Commodity 1941. This label marked rationed goods for about ten years from 1941, which could be obtained exclusively through coupons. These included products and raw materials that were only available to a limited extent during the war and post-war period, could only be imported, or were reserved for war-related purposes such as the production of uniforms.
The restrictions called up via the label are now to point out within the artwork the risky handling of antibiotics. Then as now, antibiotics are not protected as a controlled commodity and scarce resource from careless and excessive use. As a result, more and more pathogens are developing resistance to antibiotics, which were once considered miracle cures, and their effectiveness is waning. The increasing threat of multi-resistant germs such as MRSA or tuberculosis are examples of this. Against this background, how should we proceed with new methods such as CRISPR/Cas, which are also hailed as miracle cures?
Mechanistic World View
From the 18th century onward, the growing potential of the natural sciences to explain physical laws led to a mechanistic view of the world. The ability to move became an important characteristic of life, and automaton art accordingly became a tool for imitating nature, far surpassing the motionless, static works of antiquity (Bredekamp 2012, 12). By means of machines, artists attempted to copy life. Amongst the most famous works of the time was the Digesting Duck by Jacques de Vaucanson (1709-1782), which sought to imitate all vital functions of the water bird.
While René Descartes (1596-1650) was still of the opinion that humans function like a machine, the physician and philosopher Julien Offray de La Mettrie (1709-1751) went a step further and stated in 1748 that man is a machine (Reichle 2005, 119-120). The complexity of nature was no longer compared to a machine—it was understood to be mechanical (Gloy 1996, 162-184).
Penicillin
In 1928, the Scotsman Alexander Fleming, physician and bacteriologist (1881-1955), left a bacterial culture with staphylococci open in his laboratory and went on vacation. Upon his return, he noticed that the mold Penicillium notatum was grown on the unsealed Petri dish and destroyed the bacteria. Beginning in 1929, Fleming reported regularly in The British Medical Journal on penicillin, its effects, and its potential uses.
The antibiotic penicillin was first administered to a patient in 1941 and played an important role in wartime medicine. Four years later, in 1945, Alexander Fleming was awarded the Nobel Prize for Medicine and Physiology. Even then, Fleming warned in his speech at the Nobel Prize ceremony against careless use of the supposed miracle drug. Today, penicillin has lost its effectiveness when used on humans because bacteria have developed resistance.
Plant Breeding with Green Genetic Engineering
One method of genetic plant breeding involves inserting a CRISPR/Cas system containing genes with the desired traits into protoplasts of the plant. Protoplasts are individual plant cells that no longer have a cell wall. They can be specifically obtained by storing parts of a plant (for example a leaf) in a solution containing special enzymes that degrade the cell walls. In this way, the cell membrane is temporarily permeable to the entry of foreign DNA. The goal is to grow a complete plant with the desired genetic modification from the protoplasts. The growth of plants as well as the differentiation of individual cells into specific organs in the right places is controlled by hormones (phytohormones in plants) in a similar way to humans. The proportions of these phytohormones in a plant cell determine how it develops. To grow a plant from a protoplast, clearly defined amounts of the respective phytohormones are added. In this way, a cell cluster (callus) is formed first, followed by the roots and finally the germinal stalk (hypocotyl). The finished plants are genetically identical, as they are clones of the genome-edited protoplasts.
Terpenes
Terpenes are a diverse group of substances whose representatives are all built up from a variable framework of individual isoprene molecules. Due to the presence of atomic carbon double bonds in the isoprene building blocks, these have an almost unlimited number of connection possibilities, in which ring formations (cyclic terpenes), or the formation of long chains as in plastics (polyterpenes) is possible. These hydrocarbon structures form a huge family of over 8000 known different substances with varying sizes. In plants, terpenes can serve a wide variety of biological purposes, many of which are still considered largely unexplored. Most importantly, however, they play a major role in defense against pests through their widely observed antimicrobial and antiviral properties and their use as chemical messengers/pheromones. Often extracted from plants, essential oils mainly consist of a mixture of various terpenes and closely related groups of substances. The best-known application is the production of natural rubber from the milky sap of the Mexican rubber tree, which is used, for example, in the manufacture of car tires.
Text Exhibits
The context and the accompanying texts of the exhibition were developed in the winter semester 2021/22 as part of a seminar of the Institute of Art History at the Goethe University Frankfurt am Main under the direction of Prof. Dr. Viola Hildebrand-Schat and Heike Sütter. Inspired by the interdisciplinary nature of the exhibition theme, students of art history, biology, curatorial studies, and philosophy worked together in the seminar.
The source references for the wall texts and further writings, which have been compiled into a glossary, are available at www.wunderkammer-bioart.de.
Participants and authors: Lea Bligenthal, Sandra Farenburg, Katarina Haage, Tizian Holzbach, Esra Klein, Julia König, Fabian Korner, Marion Langhans, Janina Maus, Sophie Merz, Deniz Olgun, Charlotte Pignataro, Radia Soukni, Lea Weckert as well as Stavros Vraggalas, Niklas Reith and Moritz Tettenborn (Xplor e.V.) / Design and exhibition architecture: Tanja Labs (artefont)
Bibliography
Bertram, Georg W.: Kunst. Eine philosophische Einführung, Stuttgart 2005.
Bloch, Ernst: Das Prinzip Hoffnung, Frankfurt a. M. 1985.
BOKU: Universität für Bodenkultur Wien, Presseaussendungen: 01.10.2015 – BOKU-ForscherInnen klären Methanol-Stoffwechsel von Hefen auf, online unter: https://boku.ac.at/universitaetsleitung/rektorat/stabsstellen/oeffentlichkeitsarbeit/themen/presseaussendungen/presseaussendungen-2015/01102015-boku-forscherinnen-klaeren-methanol-stoffwechsel-von-hefen-auf, abgerufen am 9.2.2022.
Braddock, Alan C.: Ecocritical Art History, in: American Art, Vol. 23, No. 2 (Summer 2009). S. 24-28.
Brakensiek, Stephan: wunderkammer.internet. Der Versuch der virtuellen Welterschließung im Wissensraum ‚Sammlung‘ und ‚Computernetz‘: Überlegungen zur Verwandtschaft des assoziativen Zugriffs auf Bilder einst und jetzt, in: Kritische Berichte, 34, 2006, S. 5–16.
Brandstätter, Ursula: Erkenntnis durch Kunst. Theorie und Praxis der ästhetischen Transformation, Wien 2013.
Bredekamp, Horst: Antikensehnsucht und Maschinenglauben. Die Geschichte der Kunstkammer und die Zukunft der Kunstgeschichte, Berlin 2012.
Breedlove, Brian: Repurpose and Reuse: Artistic Perspectives on Antimicrobial Resistance, in: Journal of Emerging Infectious Diseases, 25, 2019, S. 198–199.
Bureaud, Annick: Storytelling in Art and Science. Anna Dumitriu, Make Do and Mend, in: Dies.: Perceiving, Feeling, Interpreting, Storytelling: The Art of Art-Science, 2017, online unter: http://www.annickbureaud.net/?p=1397, http://archive.olats.org/feat/05-ArtCritic-AnnaDumitriu.pdf, abgerufen am 3.3.2022.
Catts, Oron: Biological Arts/Living Arts, in: Rosi Braidotti, Maria Hlavajova (Hrsg.): Posthuman Glossary, London u. a. 2018, S. 66–68.
Davis, Joe: Microvenus, in: Art Journal, 55, 1996, S. 70–74.
Dewey, John: Kunst als Erfahrung, Frankfurt a. M. 1980.
Dumitriu et al.: Anna Dumitriu, Alex May, Özge Ata und Diethard Mattanovich: Fermenting Futures: an artistic view on yeast biotechnology, in: FEMS Yeast Research, 21, 5, 2021, S. 1–7.
Dumitriu, Anna: Website. Make Do and Mend, online unter: URL: https://www.iwm.org.uk/history/make-do-and-mend-0, abgerufen am 3.3.2022.
Ehlers, Eckart: Das Anthropozän. Die Erde im Zeitalter des Menschen, Darmstadt 2008
Fiorentini, Erna: Konvergent Divergent. Ein historischer Blick auf den Dialog von Kunst und Wissenschaft, in: Petra Gördüren (Hrsg.): Dopplereffekt. Bilder in Kunst und Wissenschaft, Köln 2010, S. 95–102.
Gloy, Karen: Das Verständnis der Natur. Die Geschichte des ganzheitlichen Denkens, München 1996.
Goethe, Johann Wolfgang von: Metamorphose der Pflanzen, Stuttgart 1980.
Haraway, Donna: Anthropocene, Capitalocene, Plantationocene, Chthulucene: Making Kin, in: Environmental Humanities, Vol. 6, 2015, S. 159-165
Holert, Tom: Künstlerische Forschung, Anatomie einer Konjunktur, in: Texte zur Kunst, 82, 2011, S. 39–62.
Horn, Eva, Bergthaller, Hannes: Anthropozän zur Einführung, Hamburg 2019
IWM: Make, Do, And Mend 1945, online unter: URL: https://www.iwm.org.uk/history/make-do-and-mend-0, abgerufen am 5.3.2022.
Jinek, Martin; Krzysztof Chylinski, Ines Fonfara, Michael Hauer, Jennifer A. Doudna, Emmanuelle Charpentier: A Programmable Dual-RNA–Guided DNA Endonuclease in Adaptive Bacterial Immunity. In: Science. Band 337, Nr. 6096, 17. August 2012, ISSN 0036-8075, S. 816–821, doi:10.1126/science.1225829, PMID 22745249.
Kant, Immanuel: Kritik der Urteilskraft, Hamburg 1993.
Knorr-Cetina, Karin: [Rezension von:] Latour, Bruno: Les Microbes. Guerre et paix suivi de Irréductions, Paris 1984, in: Social Studies of Science, 15 (1985), S. 577-586.
Kuhn, Dorothea (Hrsg.): Zur Morphologie von den Anfängen bis 1795. Ergänzungen und Erläuterungen, Abt. 1., Bd. 9 A, Weimar 1977.
Kultermann, Udo: Kleine Geschichte der Kunsttheorie, Darmstadt 1987.
Latour, Bruno: Eine neue Soziologie für einen neue Gesellschaft, Frankfurt a. M. 2014.
Maeder, Marcus: Kunst, Wissenschaft und Natur. Zur Ästhetik und Epistemologie der künstlerisch-wissenschaftlichen Naturbeobachtung, Bielefeld 2017.
Malaguti, Simone: Die Suche nach dem Glück in der deutschen Literatur. Zur Bedeutung der blauen Blume in Novalis’ „Heinrich von Ofterdingen“, in: Pandaemonium germanicum, 9, 2005, S. 207–225.
Martin, Julia: Ecocritical Art in Times of Climate Change: Tracing Ecological Relationships between Humans and Nonhumans trough the Hyperextension of Objects. London, 2015
Medina, Miguel Ángel: CRISPR Gene Editing Meets the Art World, in: The CRISPR Journal, 1, 2019, S. 317–318.
Rapp, Regine, de Lutz, Christian: Artistic Research and Ecology: Pollution, Plastic, Water, in: Ingeborg Reichle (Hrsg.): Plastic Ocean. Art and Science Responses to Marine Pollution, Berlin/Boston 2021, S. 38-57
Reichle, Ingeborg: Bio-Art: Die Kunst für das 21. Jahrhundert, in: Kunstforum International, 258, 2018, S. 44–53.
Reichle, Ingeborg: Kunst aus dem Labor, Wien/New York 2005.
Rosen, Valeska von: Nachahmung, in: Ulrich Pfisterer (Hrsg.): Metzler Lexikon Kunstwissenschaft. Ideen, Methoden, Begriffe, Stuttgart 2003, S. 240–244.
Schachinger, Sonja: Fermenting Futures 2020 Trailer, min. 7:32, online unter: https://www.youtube.com/watch?v=OidIz3PZFw0, abgerufen am 9.2.2022.
Schulz-Schaeffer, Ingo: Akteur-Netzwerk-Theorie. Zur Koevolution von Gesellschaft, Natur und Technik, in: Johannes Weyer (Hrsg.): Soziale Netzwerke. Konzepte und Methoden der sozialwissenschaftlichen Netzwerkforschung, München 2000, S. 277–300.
Seng, Eva-Maria: Konstellationsforschung als methodischer Ansatz für kooperative Forschungsumgebungen in der Kunst- und Architekturgeschichte, in: Dies., Reinhard Keil, Gudrun Oevel (Hrsg): Studiolo. Kooperative Forschungsumgebungen in den E-Humanities, Berlin 2018, S. 27–34.
Tsing, Anna: Feral Biologies. Paper for Anthropological Visions of Sustainable Futures, University College London, February 2015.
Vaage, Nora S.: What Ethics for Bio Art?, in: Nanoethics (2016) 10, S. 87–104 DOI 10.1007/s11569-016-0253-6; Eduardo Kac: Signs of life: bio art and beyond, Cambridge/Mass. 2007.
Vogel, Sabine: Leonardo im Labor. Kunst und Wissenschaft im 21. Jahrhunderts, in: Kunstforum International, 277, 2021, S. S. 52–73.
Wankerl, Barbara: Bier aus dem Reagenzglas, in: TUMcampus, Technische Universität München, 1/2013, S. 48-49, online unter: URL: https://www.tum.de/uploads/1_2013_TUMcampus.pdf, abgerufen am: 9.3.2022.
Weibel, Peter: Biotechnologie und Kunst, in: Manfred E. A. Schmutzer (Hrsg.): Technik und Gesellschaft: Symposion der technischen Universität Wien, 24.–30.8.1980, Wien/New York 1981, S. 158–169.
Yetisen, Ali K. et al.: Bio Art, in: Trends in Biotechnology, December 2015, Vol. 33, No. 12 http://dx.doi.org/10.1016/j.tibtech.2015.09.011.